Edited by Ryan Jien.
For as long as mankind has roamed the surface of this lush green planet, we've seemed always to be accompanied by a core element of life, food. Food, as we know it exists in various forms, be it legumes, grains, meat, vegetables, or fruits. Yet, it seems magical that once ingested, it can contribute to the plethora of tissues residing in this epithelial shell of us. However, just as Arthur C. Clarke once said: “Any sufficiently advanced technology is indistinguishable from magic.” Over the years, our understanding of science and physiology has slowly unraveled this wonderful mystery, allowing us to yield what we thought was witchcraft at the tips of our fingertips. In this article, I’ll be discussing how carbohydrates are processed and used in our body.
What are nutrients?
Nutrients can be simply defined as any substance that plants or animals need in order to live and grow. There are mainly two branches of nutrients — macronutrients, and micronutrients. As its name suggests, macronutrients are nutrients we need large amounts of, in contrast to micronutrients. The three groups of macronutrients — four if you consider alcohol a macronutrient — are proteins, carbohydrates, and fats (Venn, 2020). Each of these macronutrients serves a different purpose in our body and is handled differently. It is practically impossible to explain everything that happens in the body as they are ingested, as there are numerous connections, intersections, etc. (See the metabolic map below if you don’t believe me.) Therefore, I’ll only summarize the surface-level physiology of these macronutrients.

Figure 1: Metabolic Map. Image taken from Stanford Med Education. For a clearer image, visit https://metabolicpathways.stanford.edu/
What are carbohydrates?
Let’s start off with the most common macronutrient, carbohydrates. The long-standing dogma that carbohydrates are only used for energy is far outdated as it is now clear that dietary carbohydrates are a diverse group of substances with varied physiological properties. The simplest form of a carbohydrate is known as a monosaccharide. The most commonly occurring monosaccharides contain three to six carbon atoms in an unbranched single-bonded chain. The next step up is a disaccharide which consists of two monosaccharides linked together via a glycosidic bond. For example, glucose is a monosaccharide whereas sucrose is a disaccharide made up of glucose and fructose, another monosaccharide (Holesh & Martin, 2019). After disaccharides, the structure of carbohydrate molecules starts to become more complex, such as glycogen which consists of many branches of linked glucose molecules. You can take a look at the figure below to see how the structure of carbohydrate molecules gets increasingly complex.
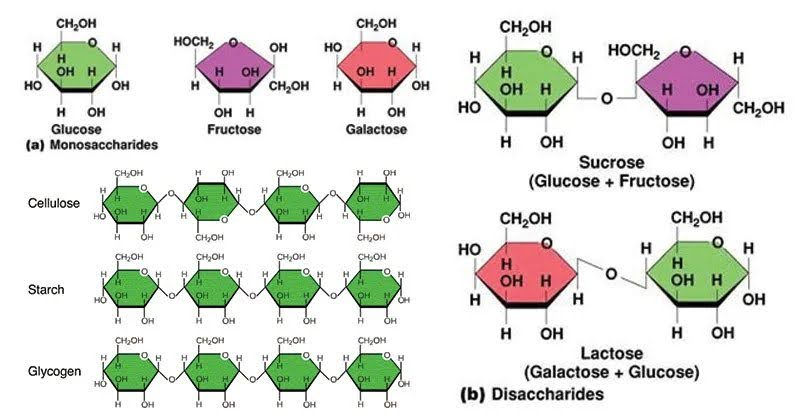
Figure 2: Diagram of mono-, di-, and polysaccharides. Image taken from Microbe Notes.
Physiological functions of carbohydrates
Due to carbohydrates’ various structures, they possess various physiological functions as opposed to only providing energy. When consuming food, the first stage of carbohydrate digestion starts in the mouth where salivary enzymes break up the large carbohydrate molecules into smaller molecules. This process is halted once the bolus of food enters the stomach, as the high acidity of our stomach’s acid prevents any salivary enzymes from working. Once the chyme from the stomach enters the duodenum, the pancreas is stimulated to secrete pancreatic enzymes that further break down the carbohydrate molecules into its monosaccharide form – abundantly glucose – where it is absorbed into the portal circulation (towards the liver) and subsequently into the systemic circulation, increasing blood glucose levels (Holesh & Martin, 2019). More complex carbohydrate molecules, those that are more highly branched take longer to digest, and have a more gradual impact on raising blood glucose levels (Wolever TM, 1990). Simple sources of carbohydrates include refined grains, sugar, high fructose corn syrup, and other more processed forms of carbohydrates, whereas complex sources of carbohydrates include unrefined grains, fiber, and starches, and are generally less processed.
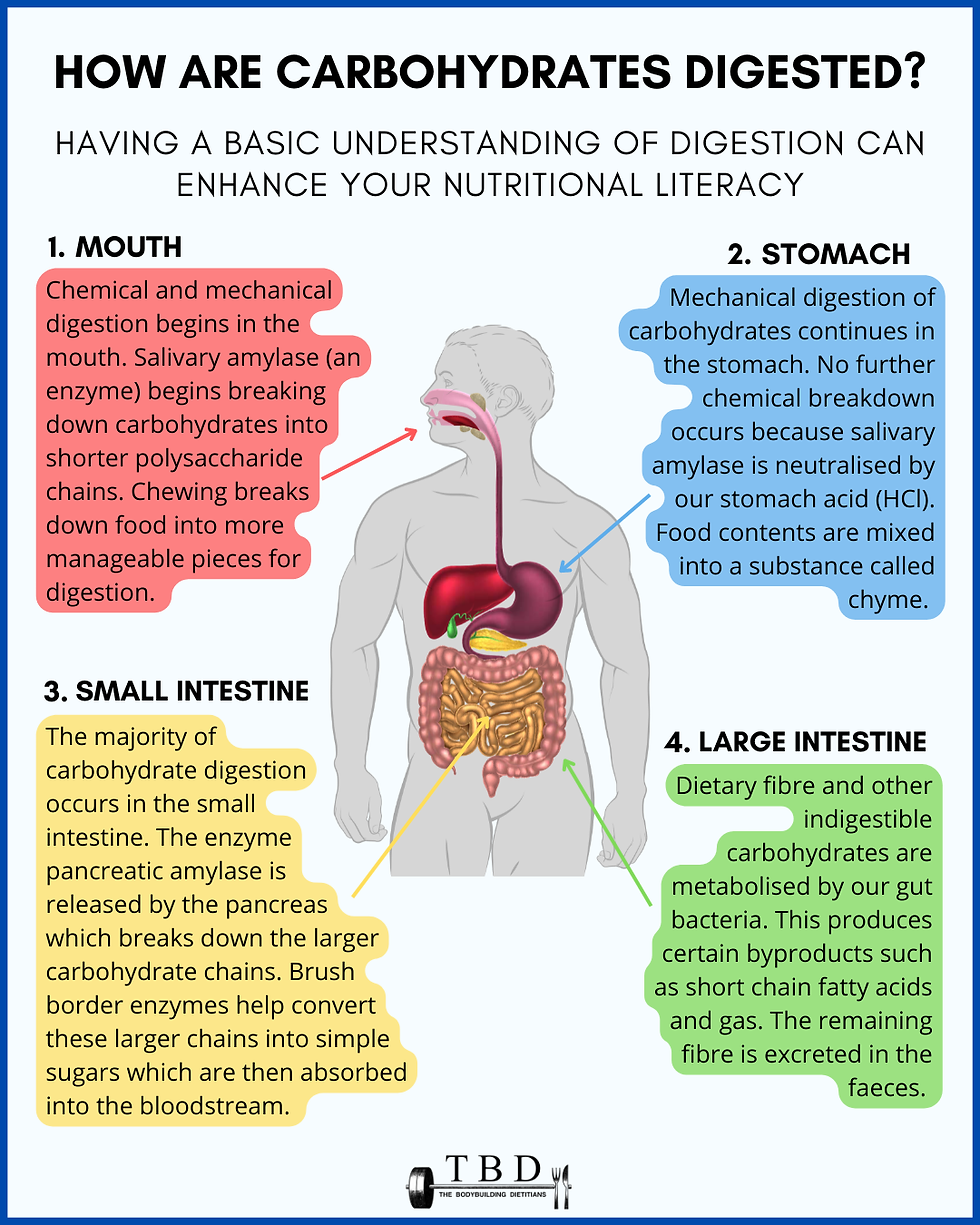
Figure 3: Carbohydrate digestion diagram. Image taken from The Bodybuilding Dietitian.
Glucose metabolism
Glucose as the dominant carbohydrate plays many roles in the body. Some of the more dominant roles include the oxidation of glucose in cells to produce energy and the regulation of blood glucose level homeostasis (Holesh & Martin, 2019). A high glucose level causes the pancreas to secrete insulin which subsequently lowers blood glucose levels (Rorsman & Renström, 2003). Besides, glucose is also the primary fuel source used by the brain, although ketones can be used as a backup fuel source as well (Mergenthaler et al., 2013). For glucose to be utilizable in most tissue cells, it needs to be transported across the cell membrane into the cytoplasm. Glucose cannot readily diffuse through because of its high molecular weight. Transport is made possible through protein carrier molecules, mainly GLUT transporters, except in the gastrointestinal tract and renal tubules, where glucose is transported along with sodium ions in a process known as co-transport. Due to the different GLUT transporters present in different organs, the rate of glucose transport into most cells is under the control of insulin (Nakrani et al., 2020).
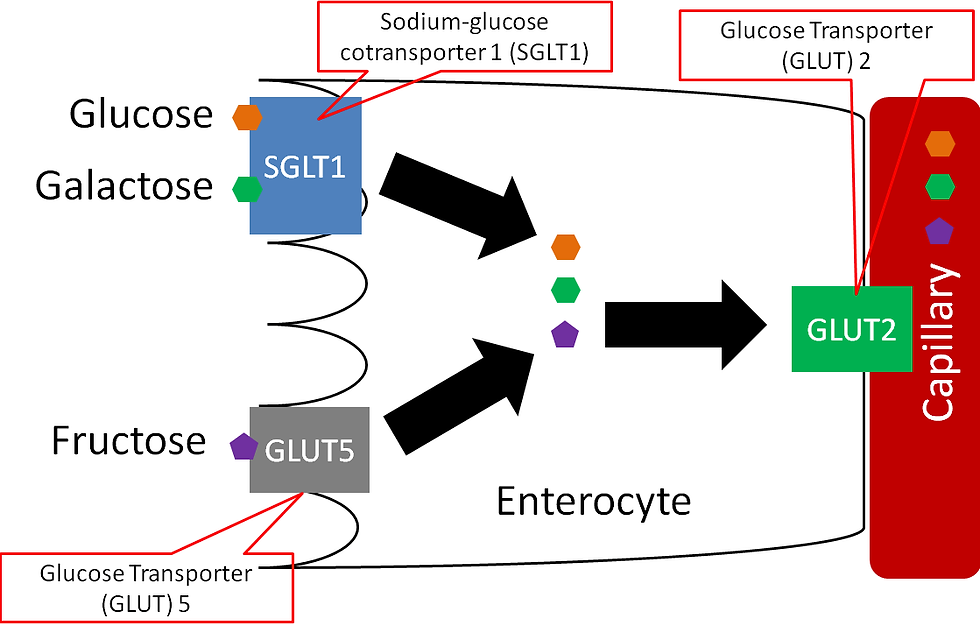
Figure 4: Monosaccharide transport in the gastrointestinal tract. Image taken from Lumen Learning.
As soon as glucose enters a cell, it is immediately attached to a phosphate group, to form glucose-6-phosphate, in order to prevent it from “escaping”. The glucose can then be used to produce energy in a process known as glycolysis (one gram of carbohydrates provides approximately 4 kcal if digested fully) or be stored in the form of glycogen in a process known as glycogenesis. Other metabolic pathways that involve glucose are the pentose-phosphate pathway, an alternative pathway of glucose oxidation that produces 5-carbon sugars usable for nucleotide synthesis, and NADPH, a molecule important in fighting off oxidative stress (Dashty, 2013).
In fact, glucose is so important that the body has two main mechanisms to increase glucose levels in times of desperation. When blood glucose becomes low (hypoglycemia), the pancreas releases glucagon while other organs such as the adrenal glands release cortisol and epinephrine. These hormones act to stimulate two processes: the first generates glucose via glycogenolysis which involves breaking down glycogen to yield glucose. The second process is gluconeogenesis which is the production of glucose from non-carbohydrate components such as amino acids (Han et al., 2016).
Metabolism of other carbohydrates
Well, what about the other monosaccharides, namely fructose and galactose? Fructose, found in fruits and most industrially sweetened food, is mainly absorbed through the GLUT5 transporter and can be then converted to a phosphorylated intermediate that can be used to generate energy in glycolysis. Unlike the oxidation of glucose, however, the phosphorylation of fructose is unregulated which has been mechanistically linked to various diseases, though human trials have mainly indicated fructose’s safety at physiological levels (Dholariya & Orrick, 2022). Galactose, obtained from the breakdown of lactose found in dairy products (which has its own problems), on the other hand, will be converted through a series of steps into glucose-6-phosphate before entering the glycolysis pathway (Dashty, 2013).
Lactose is a disaccharide composed of one molecule of glucose and one molecule of galactose. From the point of view of evolution, having the enzyme necessary to break down lactose, lactase would only be needed during infancy as babies rely on breast milk for their nutrition. Hence, in some populations, the lactase enzyme is hardly found in adults. This contributes to their inability to digest lactose, which travels all the way to the large intestine where it is fermented by our gut bacteria, producing gas that causes bloating (Daniel L. Swagerty et al., 2002). The exact reason why lactase persistence provides a selective advantage remains debated, but most agree that it involves coevolution among populations that had a high reliance on dairy products as a source of nutrition (Gerbault et al., 2011).
That’s about it for monosaccharides, but we have yet to even scratch the surface of the other carbohydrate molecules. I’ve mentioned glycogen plenty of times, so I’ll start with it. Glycogen is essentially the storage form of glucose in mammals. It can be synthesized when there is an abundance of substrate and can be broken down when glucose levels are low. However, there is a limit to how much glycogen the body can store, up to 500g. If there are any excess nutrients, not only glucose, beyond the capabilities of the body to store as glycogen, they are usually converted into fats and stored in adipose tissues in a process known as lipogenesis (Patino & Mohiuddin, 2020).
In a nutshell, carbohydrates generally have 5 metabolic pathways to choose from, glycolysis (to produce energy), gluconeogenesis (to produce glucose), glycogenolysis (to break glycogen), glycogenesis (to synthesize glycogen), and the pentose phosphate pathway (can be used for nucleic acid synthesis as well as antioxidant regeneration).
Fiber and its importance
Another interesting complex carbohydrate molecule is the group known as dietary fiber. These are part of plant material in the diet that are resistant to enzyme degradation. Unlike most sources of carbohydrates, they undergo minor digestion throughout the gastrointestinal tract and usually reach the large intestine largely intact. Dietary fiber can be subdivided into fermentable and non-fermentable fiber (García Peris & Camblor Alvarez, 1999). Fermentable fiber can be “processed” by the bacterial population in our large intestine into other metabolites, mainly short-chain fatty acids, which can positively affect various physiological functions, such as satiety, inflammation, and colon health, all while acting as a fuel source for the bacterial colony and colonocytes (Williams et al., 2019). Furthermore, the lack of fermentable fiber may produce an environment of food scarcity for the large intestines’ population of bacteria. As a result of the lack of energy availability, the bacteria may resort to fermenting proteins, which can produce toxic metabolites such as amines, thiols, sulfides, and phenols. In certain cases, the native bacteria may also ferment and use the mucosal lining of the colon, causing other problems (Desai et al., 2016). On the other hand, non-fermentable fiber cannot be fermented and used as fuel by the intestinal bacteria, but can still alter gut microbiota composition. Non-fermentable fiber can also help add bulk to the stool, which facilitates bowel movement, thus preventing constipation (Berer et al., 2018).
All in all, this piece is only a tip of the iceberg regarding carbohydrate physiology, but should serve as a primer to remind us of the importance and diverse roles of carbohydrates. As such, we should have a balanced diet, and avoid extreme ones that force us to cut out carbohydrates completely.
References:
Berer, K., Martínez, I., Walker, A., Kunkel, B., Schmitt-Kopplin, P., Walter, J., & Krishnamoorthy, G. (07 2018). Dietary non-fermentable fiber prevents autoimmune neurological disease by changing gut metabolic and immune status. Scientific Reports, 8. doi:10.1038/s41598-018-28839-3
Daniel L. Swagerty, J., Walling, A. D., & Klein, R. M. (05 2002). Lactose Intolerance. American Family Physician, 65, 1845–1851. Retrieved from https://www.aafp.org/pubs/afp/issues/2002/0501/p1845.html
Dashty, M. (10 2013). A quick look at biochemistry: Carbohydrate metabolism. Clinical Biochemistry, 46, 1339–1352. doi:10.1016/j.clinbiochem.2013.04.027
Desai, M. S., Seekatz, A. M., Koropatkin, N. M., Kamada, N., Hickey, C. A., Wolter, M., … Martens, E. C. (11 2016). A Dietary Fiber-Deprived Gut Microbiota Degrades the Colonic Mucus Barrier and Enhances Pathogen Susceptibility. Cell, 167, 1339-1353.e21. doi:10.1016/j.cell.2016.10.043
Dholariya, S. J., & Orrick, J. A. (2022). Biochemistry, Fructose Metabolism. Retrieved from https://www.ncbi.nlm.nih.gov/books/NBK576428/
García Peris, P., & Camblor Alvarez, M. (05 1999). [Dietary fiber: concept, classification and current indications]. Nutricion Hospitalaria, 14 Suppl 2, 22S-31S.
Gerbault, P., Liebert, A., Itan, Y., Powell, A., Currat, M., Burger, J., … Thomas, M. G. (03 2011). Evolution of lactase persistence: an example of human niche construction. Philosophical Transactions of the Royal Society B: Biological Sciences, 366, 863–877. doi:10.1098/rstb.2010.0268
Han, H.-S., Kang, G., Kim, J. S., Choi, B. H., & Koo, S.-H. (03 2016). Regulation of glucose metabolism from a liver-centric perspective. Experimental & Molecular Medicine, 48, e218–e218. doi:10.1038/emm.2015.122
Holesh, J. E., & Martin, A. (2019). Physiology, Carbohydrates. Retrieved from https://www.ncbi.nlm.nih.gov/books/NBK459280/
Mergenthaler, P., Lindauer, U., Dienel, G. A., & Meisel, A. (10 2013). Sugar for the brain: the Role of Glucose in Physiological and Pathological Brain Function. Trends in Neurosciences, 36, 587–597. doi:10.1016/j.tins.2013.07.001
Nakrani, M. N., Wineland, R. H., & Anjum, F. (07 2020). Physiology, Glucose Metabolism. Retrieved from https://www.ncbi.nlm.nih.gov/books/NBK560599/
Nelson, D. L., Lehninger, A. L., & Cox, M. M. (2021). Lehninger Principles of Biochemistry (8th ed.). Basingstoke.
Patino, S. C., & Mohiuddin, S. S. (2020). Biochemistry, Glycogenesis. Retrieved from https://www.ncbi.nlm.nih.gov/books/NBK549820/
Pmc, E. (2019). Europe PMC. Retrieved from https://europepmc.org/article/med/2180214
Rorsman, P., & Renstrom, E. (08 2003). Insulin granule dynamics in pancreatic beta cells. Diabetologia, 46, 1029–1045. doi:10.1007/s00125-003-1153-1
Venn, B. J. (08 2020). Macronutrients and Human Health for the 21st Century. Nutrients, 12, 2363. doi:10.3390/nu12082363
Williams, B. A., Mikkelsen, D., Flanagan, B. M., & Gidley, M. J. (05 2019). “Dietary fibre”: moving beyond the “soluble/insoluble” classification for monogastric nutrition, with an emphasis on humans and pigs. Journal of Animal Science and Biotechnology, 10. doi:10.1186/s40104-019-0350-9
Comments